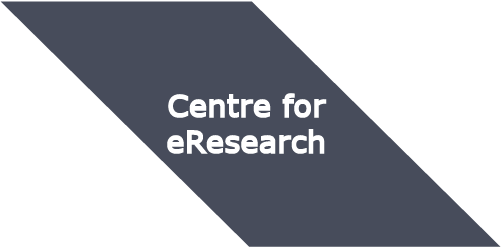
Painting the brain: multiplexed tissue labelling of human brain tissue to facilitate discoveries in neuroanatomy
Dr Helen Murray, Anatomy and Medical Imaging

Background
To understand the neurobiology of human brain diseases, anatomical studies seek to label many different tissue components across large areas, including cell populations, vasculature, and disease proteins. Many proteins must be labelled on the same tissue section to capture the complexity of human brain cytoarchitecture. However, conventional tissue labelling techniques such as immunohistochemistry (IHC) typically allow only 3–5 labels on a single tissue section. Multiplex labelling technology that generates spatial maps of large numbers of proteins can facilitate discoveries in neuroanatomy and the study of neurodegenerative diseases. Multiplexing increases the power and efficiency of neuroanatomical studies by maximising the amount of spatial protein data acquired from a single tissue section.
During my post-doctoral fellowship at the National Institutes of Health, I contributed to developing a multiplexed IHC approach for human brain tissue. This protocol allows us to label up to 80 proteins on the same slice of brain tissue, providing about 20x more data than traditional labelling methods. Since returning to New Zealand in 2020, I have been using this multiplexed IHC technique to study the brain tissue of former athletes with dementia. I also collaborate with groups within the Centre for Brain Research and across the University to use multiplex IHC for their projects.
Method
Our multiplex IHC protocol improves the throughput of conventional IHC through iterative labelling of up to 5 different antibodies per cycle, with no limitation on the number of cycles. Commercially produced primary antibodies specifically bind to proteins of interest within the tissue. Secondary antibodies conjugated to a fluorophore then bind to specific primary antibodies, resulting in a fluorescent signal where the primary-secondary antibody complex has formed. Five fluorophores are used alongside a fluorescent counterstain that labels cell nuclei. Images of the fluorescent labelling are captured using the ‘MetaSystems Vslide’ Slide Scanner in the FMHS Biomedical Imaging Research Unit. This microscope is equipped with custom filters that can spectrally separate the emission of all six fluorophores and stitch together image tiles to create whole slide images of our tissue section.
Once the images are acquired, the primary-secondary antibody complexes are stripped from the tissue, and another five primary antibodies are applied. Depending on the tissue integrity, this process can be repeated up to 10 times.
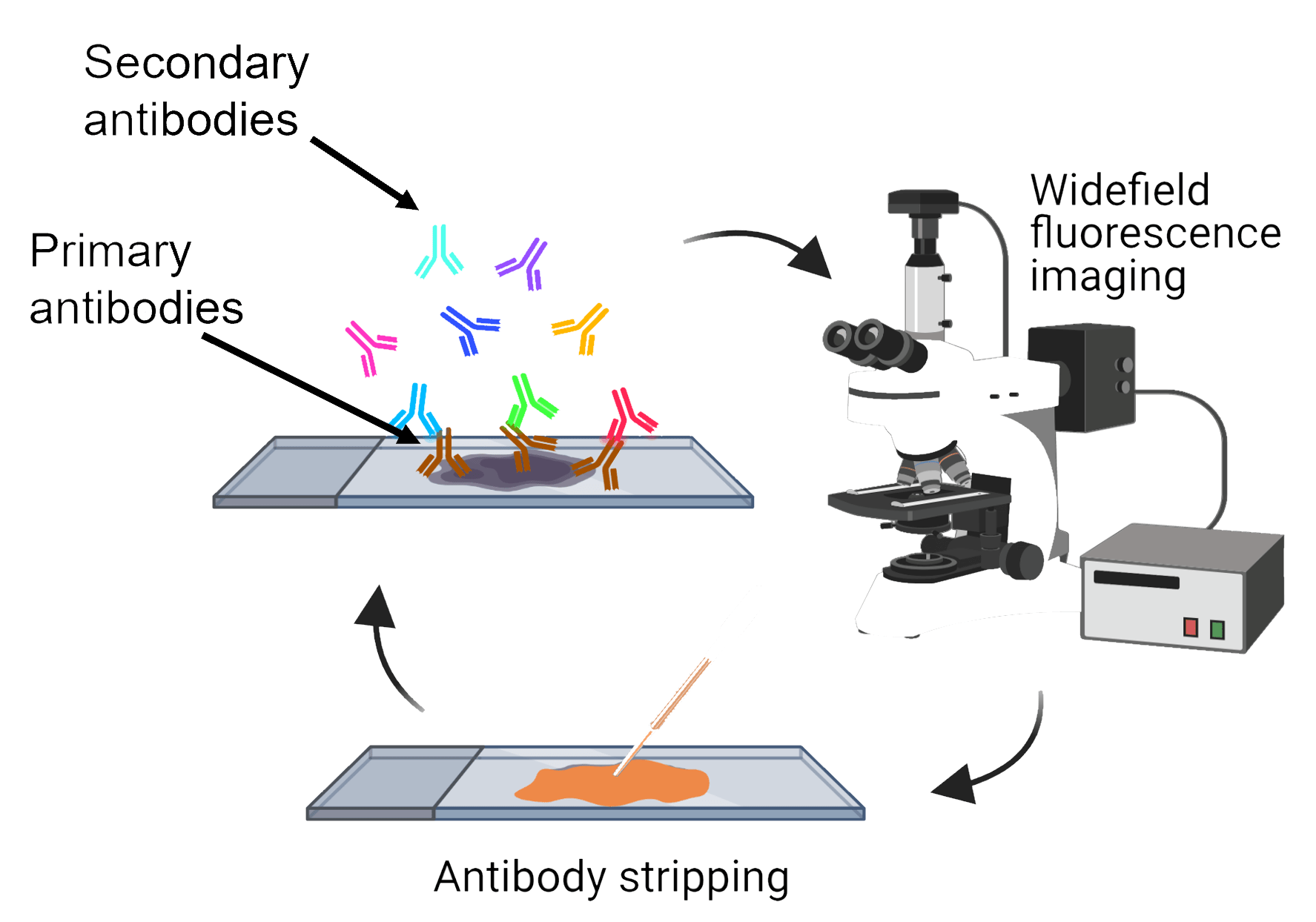
Image alignment and analysis
The production of such high-content anatomical information necessitates new image alignment and analysis tools. Our whole section images contain six channels (one for each protein of interest labelled in the round) and are 10-15 GB in size.
Once acquired, the images from each labelling round must be aligned so all proteins of interest can be visualised and analysed together. With collaborators at the Auckland Bioengineering Institute, we designed a custom registration code in Python to automatically register the nuclei images from each labelling round. We found that the registration accuracy improved when the images were pre-processed before the registration. We applied a 50-pixel rolling background subtraction and a 5-pixel median filter to the nuclei images to smooth out any nuclei staining fluctuations. The processed nuclear images were registered with each other using an AKAZE affine registration, and a transformation matrix was extracted for each image round. Subsequently, we applied this transformation matrix to all the individual images for the round. The individual images were merged into a single file and pseudo-coloured using Adobe Photoshop. An entire image dataset containing 50 proteins of interest across one whole tissue section can easily exceed 120 GB in file size.
Segmentation of image features such as individual cells or tissue layers is particularly challenging for neurological tissue due to the complex spatial arrangement of neurons, glia and their processes, and the size of human brain regions.
We use open-source image analysis software such as QuPath and ImageJ to conduct the specific analysis relevant to the biological questions we wish to explore. This analysis can be computationally expensive due to the size of the image dataset and the number of objects being analysed.
The Centre for eResearch has supported us in establishing this multiplex IHC pipeline by facilitating:
- File storage and collaboration between University of Auckland researchers using Research Drives.
Collaboration and data sharing with external collaborators using Dropbox
Computational support with virtual machines to conduct image analysis.
I look forward to future collaborations with CeR to develop more streamlined workflows for image alignment and analysis and to develop data management solutions for multiplex IHC image data.
Conclusion
By maximising the amount of information that can be collected from precious human brain tissue samples, we can ask more complex questions about how the brain is affected in disease. Multiplexed tissue labelling is already accelerating discoveries in neuroanatomy and neurodegenerative diseases, and I am excited to develop this platform at the University of Auckland.

Smyth, L. C., Murray, H. C., Hill, M., van Leeuwen, E., Highet, B., Magon, N. J., … & Hampton, M. B. (2022). Neutrophil-vascular interactions drive myeloperoxidase accumulation in the brain in Alzheimer’s disease. Acta Neuropathologica Communications, 10(1), 1-17.
Ramsden, C. E., Zamora, D., Horowitz, M. S., Jahanipour, J., Calzada, E., Li, X., … & Maric, D. (2023). ApoER2-Dab1 disruption as the origin of pTau-associated neurodegeneration in sporadic Alzheimer’s disease. Acta Neuropathologica Communications, 11(1), 1-31.
Swanson, M. E., Scotter, E. L., Smyth, L. C., Murray, H. C., Ryan, B., Turner, C., … & Curtis, M. A. (2020). Identification of a dysfunctional microglial population in human Alzheimer’s disease cortex using novel single-cell histology image analysis. Acta Neuropathologica Communications, 8, 1-16.
Swanson, M. E., Mrkela, M., Murray, H. C., Cao, M. C., Turner, C., Curtis, M. A., … & Scotter, E. L. (2023). Microglial CD68 and L-ferritin upregulation in response to phosphorylated-TDP-43 pathology in the amyotrophic lateral sclerosis brain. Acta Neuropathologica Communications, 11(1), 1-22.
Murray, H. C., Dieriks, B. V., Swanson, M. E., Anekal, P. V., Turner, C., Faull, R. L., … & Curtis, M. A. (2020). The unfolded protein response is activated in the olfactory system in Alzheimer’s disease. Acta Neuropathologica Communications, 8, 1-15.
References:
Murray, H. C., Johnson, K., Sedlock, A., Highet, B., Dieriks, B. V., Anekal, P. V., … & Maric, D. (2022). Lamina-specific immunohistochemical signatures in the olfactory bulb of healthy, Alzheimer’s and Parkinson’s disease patients. Communications Biology, 5(1), 88.
Maric, D., Jahanipour, J., Li, X. R., Singh, A., Mobiny, A., Van Nguyen, H., … & Roysam, B. (2021). Whole-brain tissue mapping toolkit using large-scale highly multiplexed immunofluorescence imaging and deep neural networks. Nature communications, 12(1), 1550.
See more case study projects

Painting the brain: multiplexed tissue labelling of human brain tissue to facilitate discoveries in neuroanatomy
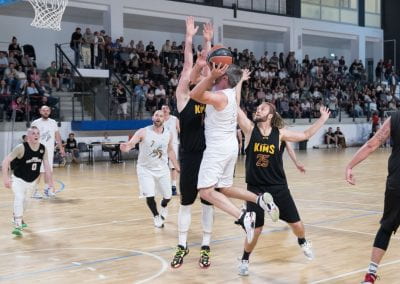
Detecting anomalous matches in professional sports: a novel approach using advanced anomaly detection techniques

Benefits of linking routine medical records to the GUiNZ longitudinal birth cohort: Childhood injury predictors

Using a virtual machine-based machine learning algorithm to obtain comprehensive behavioural information in an in vivo Alzheimer’s disease model
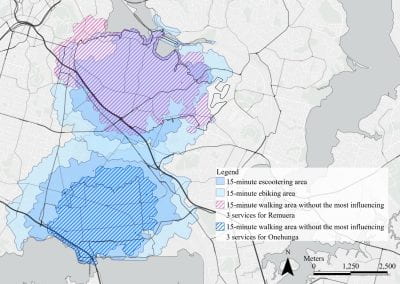
Mapping livability: the “15-minute city” concept for car-dependent districts in Auckland, New Zealand
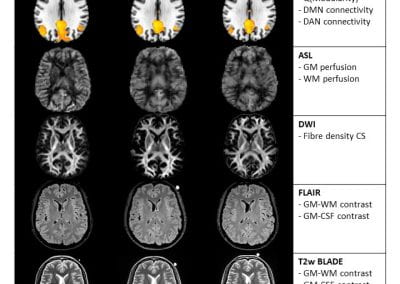
Travelling Heads – Measuring Reproducibility and Repeatability of Magnetic Resonance Imaging in Dementia

Novel Subject-Specific Method of Visualising Group Differences from Multiple DTI Metrics without Averaging
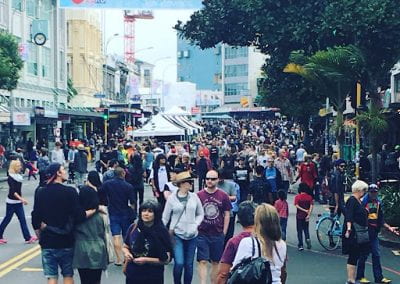
Re-assess urban spaces under COVID-19 impact: sensing Auckland social ‘hotspots’ with mobile location data
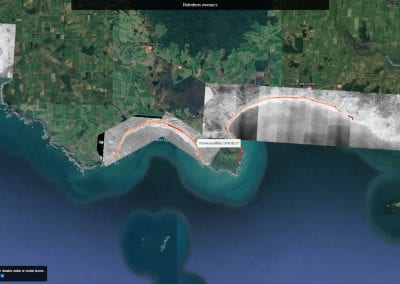
Aotearoa New Zealand’s changing coastline – Resilience to Nature’s Challenges (National Science Challenge)
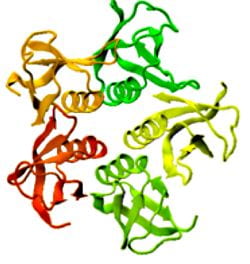
Proteins under a computational microscope: designing in-silico strategies to understand and develop molecular functionalities in Life Sciences and Engineering

Coastal image classification and nalysis based on convolutional neural betworks and pattern recognition
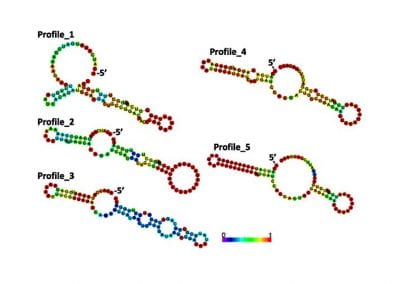
Determinants of translation efficiency in the evolutionarily-divergent protist Trichomonas vaginalis

Measuring impact of entrepreneurship activities on students’ mindset, capabilities and entrepreneurial intentions

Using Zebra Finch data and deep learning classification to identify individual bird calls from audio recordings

Automated measurement of intracranial cerebrospinal fluid volume and outcome after endovascular thrombectomy for ischemic stroke

Using simple models to explore complex dynamics: A case study of macomona liliana (wedge-shell) and nutrient variations
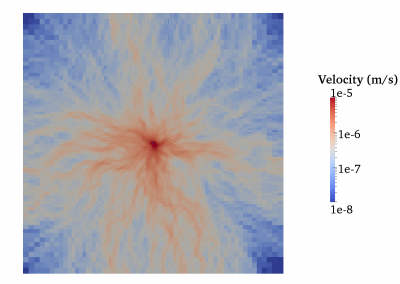
Fully coupled thermo-hydro-mechanical modelling of permeability enhancement by the finite element method
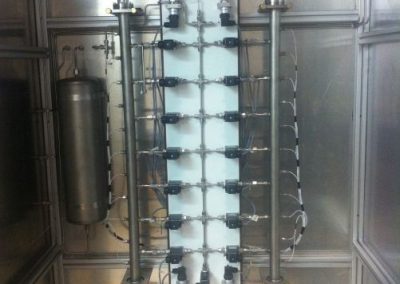
Modelling dual reflux pressure swing adsorption (DR-PSA) units for gas separation in natural gas processing

Molecular phylogenetics uses genetic data to reconstruct the evolutionary history of individuals, populations or species
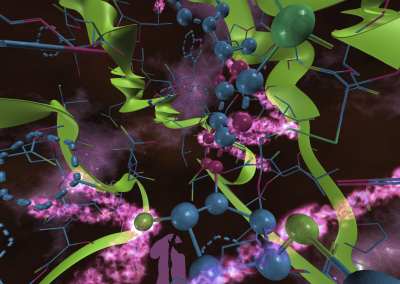
Wandering around the molecular landscape: embracing virtual reality as a research showcasing outreach and teaching tool
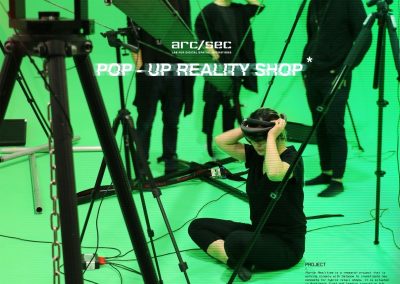