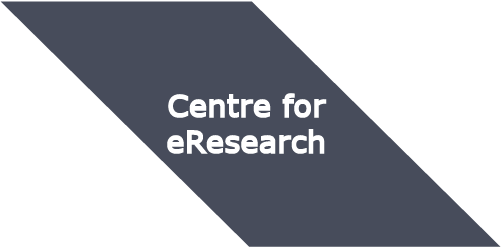
Revealing key processes in enzyme efficiency through high performance computing
Davide Mercadante, School of Chemical Science

Figure 1: Complex between Erwinia chrysanthemi pectin methylesterase (shown in yellow) and pectin decasaccharide (shown in ball and stickes). The inset shows the opposite orientations of contiguous monosaccharides along the decamer (arrow).
The fascinating ability of enzymes to act processively
Processivity is a word used to define the ability that some enzymes have to catalyze several reactions in a single binding cycle to their substrate. In other words, an enzyme binds its substrate and does not dissociate from it until more than one modification to it has been performed. Usually, processivity is exerted on substrates that have a linear conformation such as polymeric chains of DNA or polysaccharides, which need to be chemically modified in order to prompt a function.
In the plant world, there are many examples of substrates as long polymeric chains: for example the polysaccharides that are embedded in the plant cell wall, which need processive enzymatic activity in order to be dynamically re-arranged. Interestingly, plant pathogen bacteria also express enzymes that potentially have a processive activity on plant cell wall polysaccharides. One of the most interesting class of these enzymes are the bacterial Pectin methylesterases (PME), which catalyze the conversion of pectin linear regions from highly methylesterified to lowly methylesterified chains. By doing this, the chemical and physical properties of the plant cell wall are modified in a way that favors the breach of the plant cell by the pathogen. Through means of Molecular Dynamics simulations carried out using the NeSI Pan high performance computing (HPC) cluster, I investigated the ability of a bacterial PME, expressed by the plant pathogen Erwinia chrysanthemi, to slide along pectin chains after each reaction cycle. Molecular Dynamics simulations represent a gold standard in the study of molecular motions through the application of principles that describe molecular mechanics.
The study revealed for the first time the mechanism, at an atomic level, by which the protein accomplishes a processive run along the polysaccharide. It was possible to solve the puzzling problem posed by the conformation of each monosaccharide along the chain. Indeed, the functional groups that are processed by the enzyme are alternately-facing conformations suggesting that a simple sliding of the enzyme along the chain would not be sufficient to assure any processive catalysis (Figure 1).
Simulations revealed that after the first catalytic event the conformational freedom of the substrate is locally enhanced by the electrostatic repulsion between the enzyme and the freshly generated product of reaction. This repulsion promotes a rotation of the functional groups along the polysaccharides so that a new group, susceptible to catalysis, can be approached by the enzyme (Figure 2). The above described study was useful to sample both the phenomenon of monosaccharide rotations around the glycosidic bond and the subsequent sliding of the enzyme along the substrate.
These findings are remarkable with respect to the mechanism adopted by these enzymes and give insights into a strategy for processive catalysis that does not need the hydrolysis of any externally available high-energy co-factor. Instead it uses the potential already embedded in the product of reaction to yield the energy useful to catalyze more reactions along the polymer. Moreover, on a point of view more closely related to the investigated system, the discovery of this mechanism provides new evidence of how the plant cell wall is dynamically modified to favour physiological process in plants and how the infection of plant hosts by bacteria is efficiently carried out.
The sampling of substrate conformational variations crucial to bacterial infection of plant hosts will be of great help to develop new compounds able to modify pathogen defense responses in plant cells.
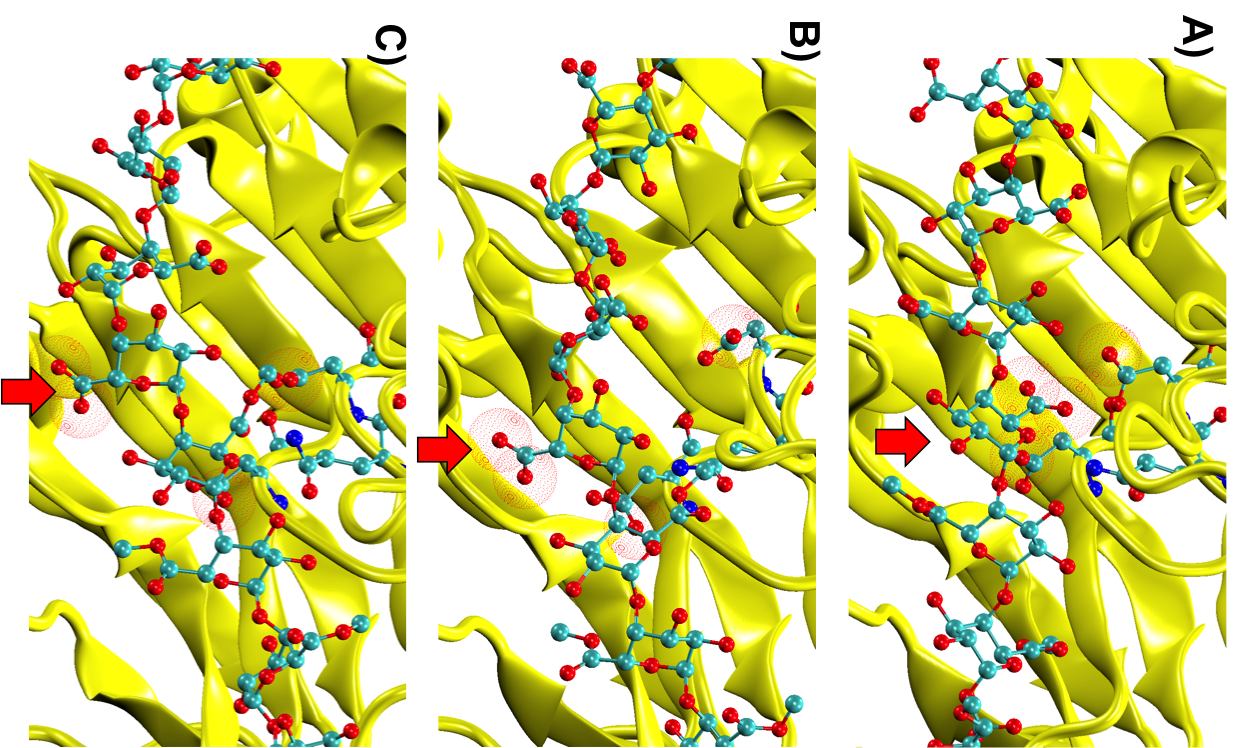
Figure 2: Conformational variations of the monosaccharide in proximity of the catalytic site of Erwinia chrysanthemi PME. The red spheres surround the atoms involved in the electrostatic repulsion between the monosaccharides and the active site (A). Such repulsion displaces the catalyzed monosaccharide out of the binding site and favours a rotation along the glycosidic bond (B). Thermal fluctuations will accomplish the sliding useful for the docking of another monosaccharide into the active site (C).
Why using high performance computing?
Molecular Dynamics simulations compute the motions of particles (which can be part of a biological or a non-biological system) by integrating, at each time-step, Newton’s equation of motion. In this equation the force acting between two particles is described as proportional to the mass of the particles and their acceleration at each time-step.
It is interesting to see that this is a very simple equation that, given all the parameters, can be easily solved by any simple calculator. Nevertheless, proteins and whatever surrounds them (water, ions, membranes or some other biological structures) are constituted by hundreds of thousands of particles (sometimes millions) that exert forces over both short (these are Lennard-Jones, hydrogen bonding and hydrophobic forces) and long-range (electrostatic forces). Computing all the forces at each time-step for hundreds of thousands or sometimes millions of particles is not an easy task although the equations involved are fairly simple. Scaling the calculations for some defined subsets of particles across different CPUs of multiple-core computers can considerably speed up the Molecular Dynamics simulations. The simulations were carried out on the Pan cluster using GROMACS 4.5.5 software as the Molecular Dynamics engine.
Reference
- Mercadante, D., Melton, L. D., Jameson, G. B., Williams, M. A. & De Simone, A. Substrate dynamics in enzyme action: rotations of monosaccharide subunits in the binding groove are essential for pectin methylesterase processivity. Biophysical journal 104, 1731-1739, doi:10.1016/j.bpj.2013.02.049 (2013).
- Mercadante, D., Melton, L. D., Jameson, G. B. & Williams, M. A. K. Processive pectin methylesterases: the role of electrostatic potential, breathing motions and bond cleavage in the rectification of Brownian motions. PLoS Computational Biology Submitted (2013).
See more case study projects

Our Voices: using innovative techniques to collect, analyse and amplify the lived experiences of young people in Aotearoa

Painting the brain: multiplexed tissue labelling of human brain tissue to facilitate discoveries in neuroanatomy
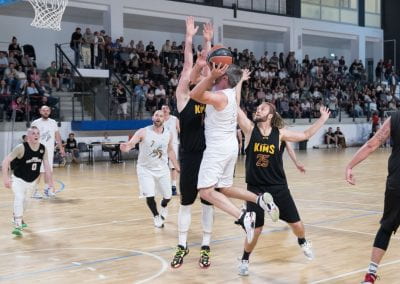
Detecting anomalous matches in professional sports: a novel approach using advanced anomaly detection techniques

Benefits of linking routine medical records to the GUiNZ longitudinal birth cohort: Childhood injury predictors

Using a virtual machine-based machine learning algorithm to obtain comprehensive behavioural information in an in vivo Alzheimer’s disease model
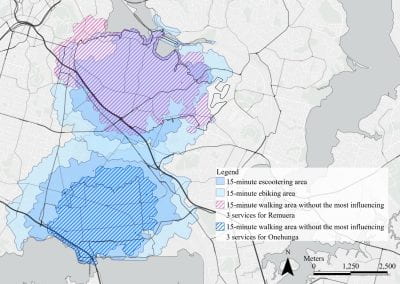
Mapping livability: the “15-minute city” concept for car-dependent districts in Auckland, New Zealand
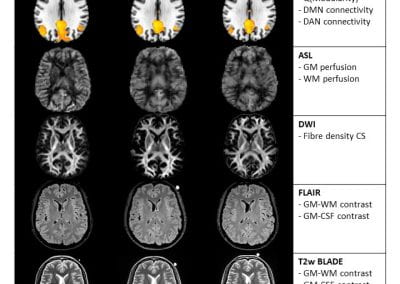
Travelling Heads – Measuring Reproducibility and Repeatability of Magnetic Resonance Imaging in Dementia

Novel Subject-Specific Method of Visualising Group Differences from Multiple DTI Metrics without Averaging
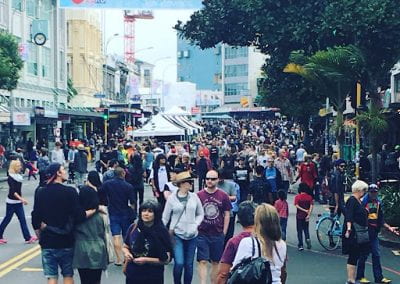
Re-assess urban spaces under COVID-19 impact: sensing Auckland social ‘hotspots’ with mobile location data
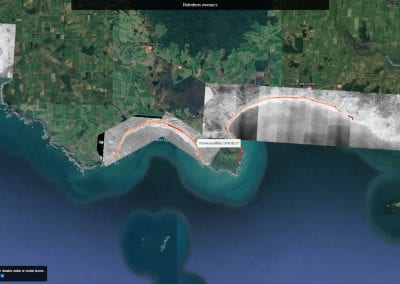
Aotearoa New Zealand’s changing coastline – Resilience to Nature’s Challenges (National Science Challenge)
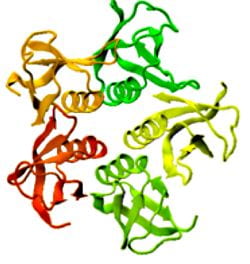
Proteins under a computational microscope: designing in-silico strategies to understand and develop molecular functionalities in Life Sciences and Engineering

Coastal image classification and nalysis based on convolutional neural betworks and pattern recognition
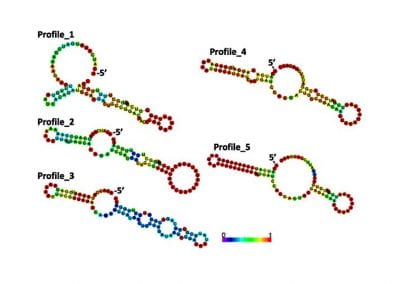
Determinants of translation efficiency in the evolutionarily-divergent protist Trichomonas vaginalis

Measuring impact of entrepreneurship activities on students’ mindset, capabilities and entrepreneurial intentions

Using Zebra Finch data and deep learning classification to identify individual bird calls from audio recordings

Automated measurement of intracranial cerebrospinal fluid volume and outcome after endovascular thrombectomy for ischemic stroke

Using simple models to explore complex dynamics: A case study of macomona liliana (wedge-shell) and nutrient variations
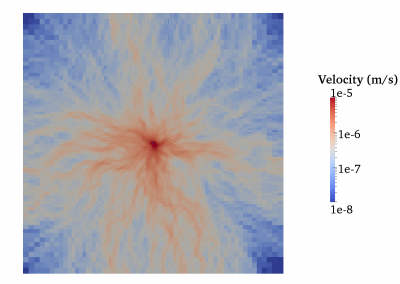
Fully coupled thermo-hydro-mechanical modelling of permeability enhancement by the finite element method
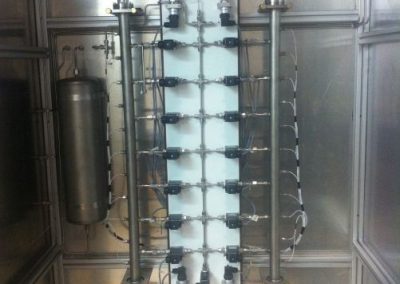
Modelling dual reflux pressure swing adsorption (DR-PSA) units for gas separation in natural gas processing

Molecular phylogenetics uses genetic data to reconstruct the evolutionary history of individuals, populations or species
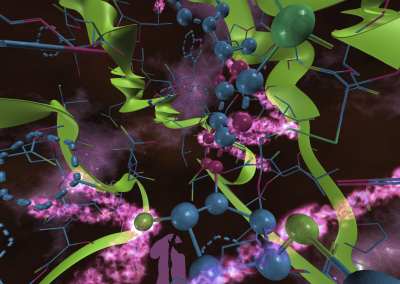
Wandering around the molecular landscape: embracing virtual reality as a research showcasing outreach and teaching tool
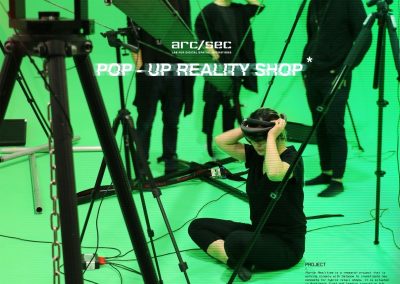