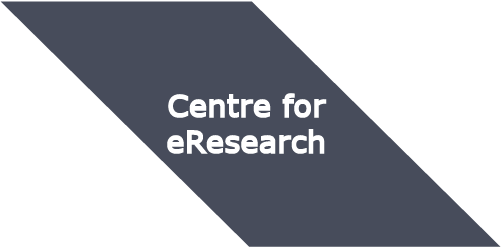
Testing what cosmic inflation really predicts
Layne Price, Department of Physics
Overview
A period of accelerated expansion in the early universe has recently received outstanding support from precision measurements of the cosmic microwave background. This “inflationary” epoch occurs at high energy scales where the exact behaviour of physics is uncertain. We focus on the numerical exploration of the dynamics of early universe models that have many degrees of freedom, which generically arise in high energy theories such as supersymmetry or string theory.
Inflation in the era of precision cosmology
Inflation is an early period in the universe’s history when the cosmological expansion accelerated. Paradoxically, this acceleration helps to explain both the general smoothness of the cosmic microwave background (CMB) and the statistics for the tiny hot and cold spots that cause the CMB to deviate from perfect homogeneity. Inflation has recently received significant observational support from measurements of the temperature and polarization of the CMB, which were taken by the space-based telescopes WMAP and Planck, as well as the ground-based telescope BICEP2.
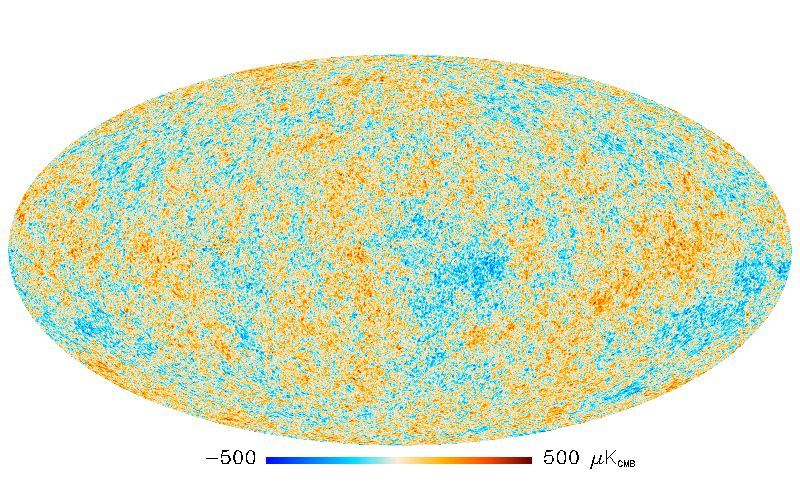
The hot and cold spots in the cosmic microwave background (CMB) as measured by the space-based telescope Planck. The CMB is at an average temperature of 2.7K and these fluctuations occur only at the level of micro-K, making a high resolution picture like this a true experimental achievement. [arXiv:1303.5062]
Inflation operates at energy scales that are vastly higher than any other known physical process, with typical energies that are 14 orders of magnitude higher than what can be obtained at the Large Hadron Collider at CERN. Before these recent developments in precision cosmology, the exact physics at these scales was exclusively the playground of speculative ideas on quantum gravity, string theory, and supersymmetry. While simple inflationary models seem to be able to reproduce the CMB data, only recently have people begun to explore the consequences of inflationary models that incorporate features generally expected to be present in high energy particle physics.

This shows the scalar field potential energy density V as a function of one scalar quantum field (left) or two fields (right). The red dots indicate some possible initial positions for the fields, while the black lines show the paths the fields would take during inflation. With one field you can only go one way down the potential; all initial conditions give the same outcome. With more than one field the paths are different for different initial conditions and each of these predict slightly different statistics for the CMB.
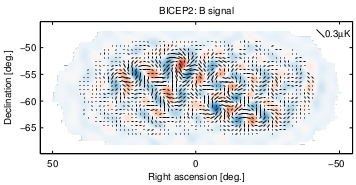
The detection of the B-mode polarization of the CMB by the BICEP2 telescope in Antarctica. The simplest explanation for this polarization spectrum is due to gravitational waves in the early universe, with an amplitude consistent with what is predicted by the simplest inflationary models. [arXiv:1403.3985]
The role of high performance computing
In our work we have focused on exploring the generic predictions of more complicated particle physics models of inflation that incorporate many active degrees of freedom. We have developed a series of numerical tools that are able to perform efficient simulations of the relevant physical processes at work in the early universe. Deriving a complete picture of what one of these models predicts requires extensive sampling of a high dimensional parameter space, the numerical evaluation of a coupled system with hundreds of nonlinear differential equations, the simulation of the plasma dynamics that generate the CMB, and the construction and evaluation of observationally relevant statistics.
We have leveraged the computational resources of the Pan cluster to perform MPI-parallelized simulations of millions of possible early universe scenarios. We have focused extensively on the role that the universe’s initial conditions play in changing the end-state of a given universe. This allows us to gauge how special our particular universe is amongst the range of possibilities. We have shown that due to non-linear interactions in the early universe that the initial conditions do not have to be fine-tuned (JCAP 1307 (2013) 027; arXiv:1304.4244) and that they have only a very minor impact on the CMB statistics (Phys.Rev.Lett. 112 (2014) 161302; arXiv:1312.4035), greatly simplifying the physics. These results lend support to the fact that inflation does not necessarily require a fine-tuned initial state in order to produce a CMB that is statistically similar to ours. While we have many international collaborators, the New Zealand computing assets and the support available from the Centre for eResearch have enabled us to complete our research quickly and with more resolution than we expected.
Future work
The next step in this project is to use lattice simulations of partial differential equations to evaluate the role of spatial gradients in the initial conditions. We will also perform a complete statistical analysis of the Planck and BICEP2 data for these more complicated inflation models by calculating the Bayesian evidence and posterior probabilities. This will require extensive numerical work where we have to explore the entire allowed parameter space and weight each possible set of parameters against how likely it is that it could reproduce our CMB. The work that we have done to-date provides a series of significant first steps toward this more ambitious goal.
See more case study projects

Our Voices: using innovative techniques to collect, analyse and amplify the lived experiences of young people in Aotearoa

Painting the brain: multiplexed tissue labelling of human brain tissue to facilitate discoveries in neuroanatomy
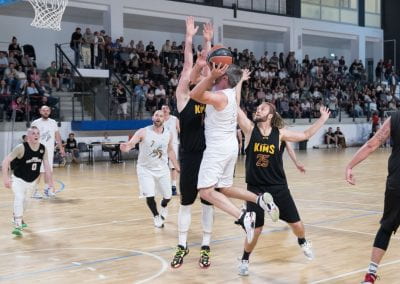
Detecting anomalous matches in professional sports: a novel approach using advanced anomaly detection techniques

Benefits of linking routine medical records to the GUiNZ longitudinal birth cohort: Childhood injury predictors

Using a virtual machine-based machine learning algorithm to obtain comprehensive behavioural information in an in vivo Alzheimer’s disease model
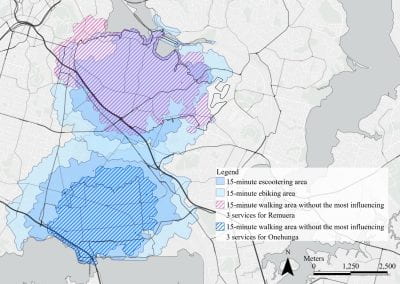
Mapping livability: the “15-minute city” concept for car-dependent districts in Auckland, New Zealand
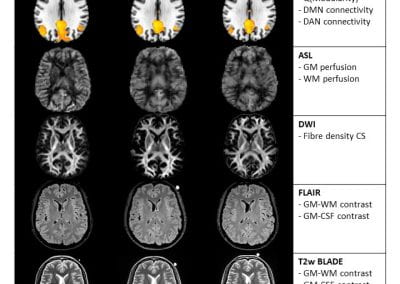
Travelling Heads – Measuring Reproducibility and Repeatability of Magnetic Resonance Imaging in Dementia

Novel Subject-Specific Method of Visualising Group Differences from Multiple DTI Metrics without Averaging
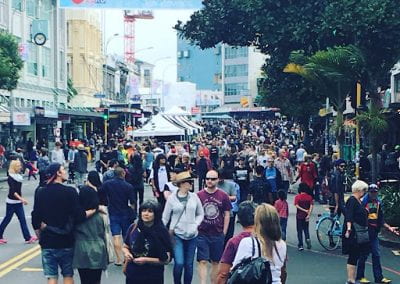
Re-assess urban spaces under COVID-19 impact: sensing Auckland social ‘hotspots’ with mobile location data
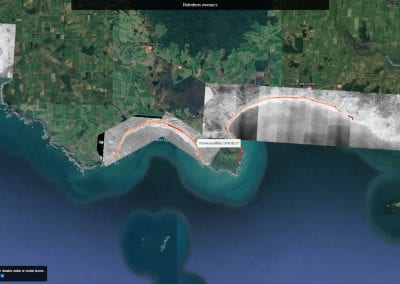
Aotearoa New Zealand’s changing coastline – Resilience to Nature’s Challenges (National Science Challenge)
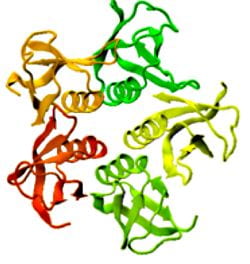
Proteins under a computational microscope: designing in-silico strategies to understand and develop molecular functionalities in Life Sciences and Engineering

Coastal image classification and nalysis based on convolutional neural betworks and pattern recognition
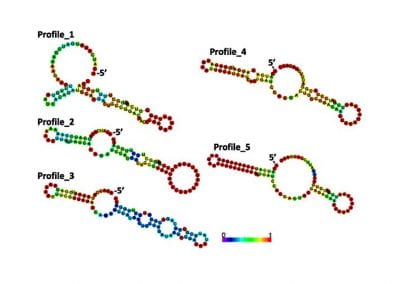
Determinants of translation efficiency in the evolutionarily-divergent protist Trichomonas vaginalis

Measuring impact of entrepreneurship activities on students’ mindset, capabilities and entrepreneurial intentions

Using Zebra Finch data and deep learning classification to identify individual bird calls from audio recordings

Automated measurement of intracranial cerebrospinal fluid volume and outcome after endovascular thrombectomy for ischemic stroke

Using simple models to explore complex dynamics: A case study of macomona liliana (wedge-shell) and nutrient variations
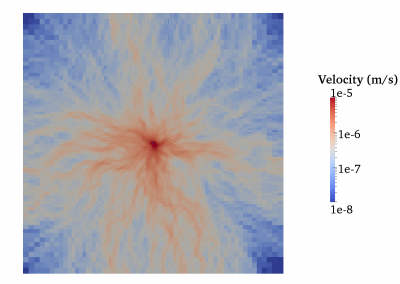
Fully coupled thermo-hydro-mechanical modelling of permeability enhancement by the finite element method
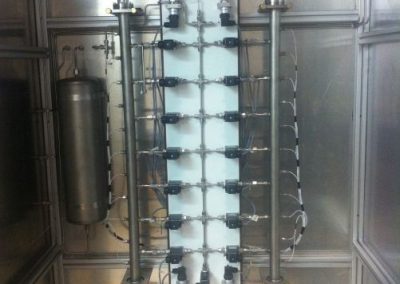
Modelling dual reflux pressure swing adsorption (DR-PSA) units for gas separation in natural gas processing

Molecular phylogenetics uses genetic data to reconstruct the evolutionary history of individuals, populations or species
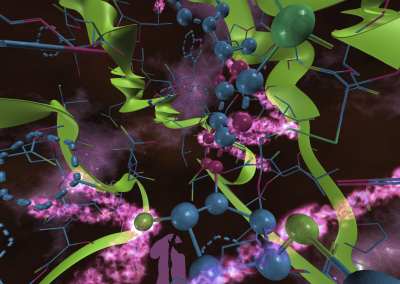
Wandering around the molecular landscape: embracing virtual reality as a research showcasing outreach and teaching tool
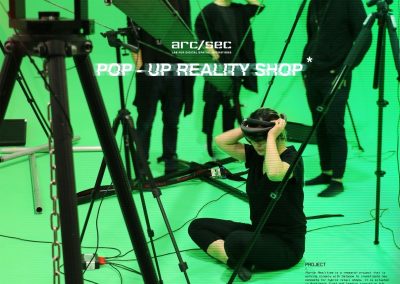